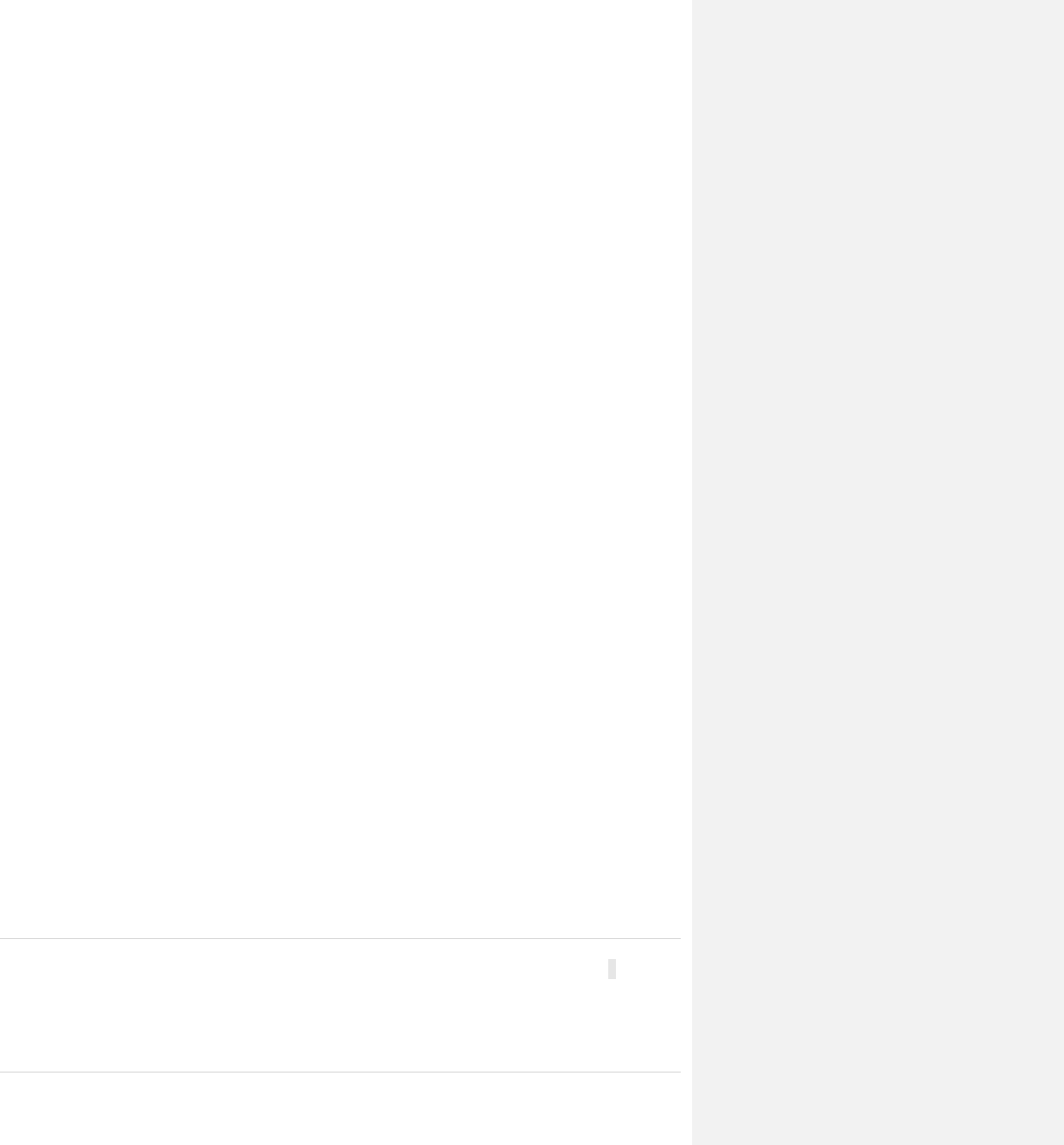
6 | P a g e
1
Kwon, HS., Ryu, M.H. & Carlsten, C. Ultrafine particles: unique physicochemical properties relevant to health
and disease. Exp Mol Med 52, 318–328 (2020). https://doi.org/10.1038/s12276-020-0405-1
2
Ostro, B et. al, “Associations of Mortality with Long-Term Exposures to Fine and Ultrafine Particles, Species
and Sources: Results from the California Teachers Study Cohort,” Environmental Health Perspectives 123(6),
June 2015.
Background
During the current year, both ultrafine particles, measured as particle count, and black carbon,
measured in nanograms per cubic meter (ng/cm
3
), were taken in one-minute intervals. This
changed from previous years when particle count was measured in 10 second intervals and
greatly reduces the volume of measurements taken, allowing for easier data processing and
analysis. There is no loss in useable data from this change because the 10 second interval data
was too variable to use without averaging it by minute. For the current analysis, both particle
count and black carbon levels were averaged into one day intervals and compared against the
daily weather data. A daily timeframe was chosen because health data are available on a daily
timeframe. Though not included in this report, future analyses may examine any correlation
between health outcomes in nearby communities and air pollution levels.
Ultrafine particles, particles less than 100 nanometers (nm) in diameter, are associated with
cardiovascular disease and other health concerns and may be more toxic than more commonly
measured larger particles, such as fine particles less than 2.5 micrometers (PM
2.5
). Ultrafine
particles are primarily emitted by combustion. Their concentration is elevated near pollution
sources and decreases quickly with time and distance, due to dispersal and a combination of
evaporation of small particles and condensation onto larger particles. These processes increase
particle size while reducing particle count. Ultrafine particles are thus representative of local
pollution sources and not suitable for evaluating regional pollution. Because of the variability in
their level over short distances, it isn’t feasible to establish standards for levels of ultrafine
particles and it has been challenging to evaluate the associated health effects. However, they
are also easier to associate with specific sources. The measurement of ultrafine particles in the
current study provides an opportunity to better understand the conditions leading to their
production and may allow future investigations into associated health effects.
Although it is difficult to distinguish the effects of ultrafine particles from other pollutants that
they typically cooccur with, there is increasing evidence to suggest that short-term ultrafine
particle exposure causes respiratory and cardiovascular health effects. Toxic substances tend to
be concentrated on ultrafine particles and more bioavailable, and thus may be more likely to
cause toxicity than larger particles.
1
Due to their very small size, ultrafine particles, when
inhaled, can travel deep into the respiratory tract and pass across membranes in the body that
would block the movement of larger particles. A 2015 study by the California EPA demonstrated
that long-term exposure to ultrafine particles contributes to heart disease mortality. Certain
constituents of ultrafine particles, including copper, iron, other metal, and elemental carbon
(soot) were strongly associated with death from heart attacks.
2